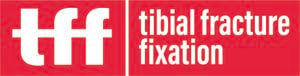
This module provides data-driven, gender-specific, easy to print, labor-saving, environmentally friendly, hygienic, and cruelty-free bone simulation models that are not made with natural rubber latex, are designed with safety features to protect users, and can be locally reproduced to provide the highest fidelity, standardized orthopedic surgical simulation training at the lowest cost.
Innovative Design[edit | edit source]
This module provides data-driven, gender-specific, easy to print, labor-saving, environmentally friendly, hygienic, and cruelty-free bone simulation models that are not made with natural rubber latex, are designed with safety features to protect users, and can be locally reproduced to provide the highest fidelity orthopedic surgical simulation training at the lowest cost.
Safety By Design[edit | edit source]
Our team has prioritized safety in the design of our simulators.
All of the module's 3D printed bone models have a vise attachment to allow the user to secure the model inside a standard vise clamp to maximize safety during simulation training. Although using a vise clamp is more costly, it is standard training practice for bone models to be secured inside a vise clamp to maximize safety during bicortical drilling and fracture fixation simulation training.[1][2][3][4] Based on our clinical experience and user testing, we do not recommend having an assistant hold a bone model during drilling training because this exposes the assistant to potential injury. There is always a risk that the drill bit may slip when drilling through the bone simulation model during training.[5]
This training module instructs all learners to wear proper protective equipment (protective eyewear and gloves) during the simulation skills training in accordance with "The ten best practices for healthcare simulation safety" as listed on the Foundation for Healthcare Simulation Safety website.[6]
High Fidelity[edit | edit source]
To maximize learning efficiency and minimize simulator overall costs and assembly time, we provide high fidelity, standardized bone simulation models for basic and advanced simulation training instead of having the learner progress through a range of homemade (DIY) simulators of varying and increasing fidelity.
The 3D printed bone models accurately simulate bone length and diameter, external contour, cross-sectional shape, bicortical anatomy, cortical hardness, cancellous bone porosity, and microstructure, and far cortex thickness for both genders at tibial shaft fracture drilling sites for modular external fixation.[7][8][9][10][11][12][13][14][15][16]
Cortical thickness varies depending on gender, age, and regions in the tibia.[16] In LMICs, tibial fractures predominantly occur in young adults (< 40 years) with a male to female patient ratio of 3:1.[17] The 3D printed bone models are designed to accurately simulate far cortex thickness values for young (< 50 years), healthy (BMI < 30) adult males and females at tibial diaphysis drilling sites for modular external fixation.[16]
During bicortical drilling skills training, the learner adjusts the applied drilling force to minimize plunge depth.[18] Drilling forces are influenced by cortical thickness, bone site, patient age, and bone mineral density.[19] To minimize the risk of teaching anti-skills (excessive or inadequate drilling forces), the high fidelity 3D printed bone models are made of plastic with similar hardness to human cortical bone and are designed to accurately simulate the age, gender, and bone site-specific cortical thickness values and cancellous bone porosity for the target patient population.[10][11][12][16][17]
A simulator without augmented (extrinsic) feedback is a higher fidelity simulator than a simulator with augmented feedback because this more accurately reflects real clinical scenarios. Thus, we removed the augmented feedback feature for plunge detection from the Tibial Shaft Simulator and Tibial Shaft Transverse Fracture Simulator to provide higher fidelity bicortical drilling and modular external fixation skills training to learners.
Easy to Manufacture[edit | edit source]
3D printing technology empowers local, labor-saving, and automated reproduction of high fidelity bone simulation models to permit standardized, high quality, self-assessed simulation training in LMICs. Using our high fidelity 3D printed bone models substantially simplifies the simulator build, minimizes the simulator assembly time, and accelerates the learning process for greater convenience for the learner.
High fidelity 3D printed surgical simulation models are typically made on expensive ($300,000 USD), closed system printers that use costly, proprietary filaments ($302.50 - $432.26/kg USD) and software and require a team of highly trained professionals to operate and maintain.[20][21] These high priced 3D printed anatomic models generally require time-consuming, laborious, and multi-step digital file preparation and significant post-processing of the 3D printed model before use by the practitioner.[20][21][22][23]
All of the module's 3D printed bone simulation models are open source and can be locally reproduced on open-source, open filament, user friendly, single extruder desktop 3D printers that print polylactic acid (PLA), a low-cost and easy to print plastic.[24][25][26][27][28][29]
To minimize material, equipment and labor costs during production, all of the module's 3D printed bone models are designed to:
- print without support material, rafts or brims
- require no cleaning, sanding, gluing, priming, painting, dipping, coating, smoothing, polishing, or any post-processing
- not require any non-3D printed parts, and
- be ready for use right out of the 3D printer.[30]
The Tibial Shaft Simulator and Tibial Shaft Transverse Fracture Simulator are easy and quick to assemble and do not require any tools, specialized equipment, technical expertise, or time-consuming preparation to build, install, operate and maintain these simulators within the intended place of use.
Lower Cost[edit | edit source]
The 3D Printed Adult Tibial Bone Models can be locally reproduced to provide the highest fidelity simulators at the lowest cost for orthopedic surgical simulation training. The costs of the 3D Printed Adult Tibial Bone Models will vary depending on the region's 3D printing organizations, and locally available brands of filament.
In Canada, a 1.0 kg roll of white PLA costs $17.95 CAD which is equal to about 1.4¢ USD per gram.[31] The Tibial Shaft Simulator (3D Printed Adult Female Tibial Bone Model #1) weighs 138.57 grams and the total filament cost in Canada is $1.95 USD. The Tibial Shaft Transverse Fracture Simulator (3D Printed Adult Female Tibial Bone Models # 2 and # 3) weighs 332.86 grams and the total filament cost in Canada is $4.69 USD.
In Nigeria, one 750 gram roll of Ultimaker White PLA filament (Shore Hardness 83D) costs €33 Euros which is equal to about 5¢ USD per gram.[10][32] The total filament cost of the Tibial Shaft Simulator (3D Printed Adult Female Tibial Bone Model #1) in Nigeria can be $6.88 USD or less. The total filament cost of the Tibial Shaft Transverse Fracture Simulator (3D Printed Adult Female Tibial Bone Models # 2 and # 3) in Nigeria can be $16.53 USD or less.
The benefits of 3D printing the Tibial Shaft Transverse Fracture Simulator locally in Nigeria for modular external fixation skills training are that the 3D printer filament costs are over 3 times cheaper and the production time is over 33 times faster than purchasing a comparable artificial bone product that is imported from abroad, and the 3D printer filament costs are over 9 times cheaper than acquiring a human cadaveric tibia prepared by a local university anatomy lab.[32][33][34] By obtaining locally made 3D printed bone models for modular external fixation skills training, the learner also supports the local economy while saving on customs dues, processing fees, and international shipping costs that would be incurred when using artificial bone products that are not made locally.
Tibial Shaft Transverse Fracture Simulator
(3D Printed Adult Female Tibial Bone Models #2 and #3) |
Sawbones Tibia, Plastic Cortical Shell, Left
(SKU:1104-9)[33] |
Human Cadaveric Tibia
(Prepared by an University Anatomy Lab in Nigeria)[34] | |
---|---|---|---|
Bone Simulator Features | 3D printed, biorenewable plastic anatomic bone models are made with a rigid plastic shell and inner cancellous material. | Plastic cortical shell models are made of a rigid plastic shell with inner cancellous material. |
|
Fracture Simulation | Simulates a transverse mid-shaft fracture of the tibia for modular external fixation training. | Requires additional preparation by user to simulate a fracture. | Requires additional preparation to simulate a fracture. |
Fracture Encapsulation | Encapsulates transverse fracture with cellophane. | Does not encapsulate or re-attach fracture. | No. This would incur additional preparation and storage fees. |
Vise Attachment | Contains a vise attachment to safely secure the model inside a standard vise clamp. | Does not contain a vise attachment. | Does not contain a vise attachment. |
Bone Simulator Dimensions | Tibia with an overall length of 41 cm. | Tibia with an overall length of 42 cm. | Varies. |
Unit Cost | $16.53 USD[32] | $53.50 USD | $150.00 USD |
Production Time | 15 hours 8 minutes (when Adult Female Tibial Bone Models #2 and #3 are printed consecutively). | Ready to ship in 21 days or more. | Depends on local availability of cadaver specimens which is difficult to predict. |
Noteː A product comparison was not made with the:
- Sawbones Tibia, Solid Foam, Large ($16.00 USD) because this model simulates the intramedullary canal but not cancellous bone, and the foam material does not simulate the hardness of cortical bone and thus, could foster anti-skills, and
- Sawbones Cylinder with Encapsulated Oblique Fracture ($37.50 USD) because the hollow short fiber reinforced epoxy cylinder does not have anatomic features that make it suitable for modular external fixation training and does not appear to have adequate length to properly simulate an adult tibial midshaft fracture for modular external fixation training which requires the placement of widely spaced pins in each fracture fragment.[35][36][37]
The benefits of 3D printing the Tibial Shaft Simulator locally in Nigeria for bicortical drilling skills training are that the 3D printer filament costs are 12 times cheaper and the production time is 84 times faster than purchasing a comparable artificial bone cylinder product that is imported from abroad.[32][38] By obtaining locally made 3D printed bone models for bicortical drilling skills training, the learner also supports the local economy while saving on customs dues, processing fees, and international shipping costs that would be incurred when using artificial bone products that are not made locally.
Tibial Shaft Simulator
(3D Printed Adult Female Tibial Bone Model #1) |
Sawbones Composite Cylinder
(SKU:3403-7)[38] | |
---|---|---|
Bone Simulator Features | Anatomic model that simulates mid-diaphyseal tibial bone for bicortical drilling skills training in preparation for modular external fixation training of an open tibial shaft transverse fracture. | Cylinder that simulates mid-diaphyseal bone for fracture fixation testing. |
Bone Simulator Materials | 3D printed, biorenewable plastic anatomic bone models are made with a rigid plastic shell and inner cancellous material. | Hollow short fiber reinforced epoxy cylinder. Customized cellular rigid polyurethane foam filling available upon request. |
Vise Attachment | Contains a vise attachment to safely secure the model inside a standard vise clamp. | Does not contain a vise attachment. |
Bone Simulator Dimensions | Variable outer diameter (including 40 mm) x 6.2 mm wall thickness x 203.05 mm length. | 40 mm outer diameter x 6 mm wall thickness x 500 mm length. |
Unit Cost | $6.88 USD per model[32] | $78.78 USD (original $194.00 USD pricing adjusted for model length of 203.05 mm)[38][39] |
Production Time | 6 hours and 2 minutes | Ready to ship in 21 days or more[38] |
Not Made With Natural Rubber Latex[edit | edit source]
Instead of using conventional rubber latex bands glued to re-attach artificial bone fracture fragments, the Tibial Shaft Transverse Fracture Simulator uses inexpensive, locally available, and easy to apply clear cellophane to encapsulate displaced fracture fragments in a simulated soft-tissue envelope.[40] Using cellophane, a material that is not made with natural rubber latex, reduces the risk of triggering latex sensitivities and potentially life-threatening allergic reactions in healthcare workers.[41]
Eco-Friendly[edit | edit source]
All of the module's 3D printed bone simulation models are made from polylactic acid (PLA), a biorenewable, biodegradable, and minimally off-gassing thermoplastic.[42][43][44][45][46][47]
Cruelty-Free[edit | edit source]
Our 3D printed bone models provide a higher fidelity, hygienic, and humane training alternative to using live animal models or mammal cadaveric bones which makes them suitable for the 1.2 billion followers of Hinduism, over 520 million followers of Buddhism, 4.5 million followers of Jainism, and ethical vegans who refrain from using any animal products.[48][49][50][51]
No animal should be harmed from using this training module in accordance with the principles of ahimsa (non-violence or non-injury to all living beings), no killing of any living being under the Right Action Factor of The Eightfold Path of Buddhism, and the recognition of consciousness of all mammals outlined in The Cambridge Declaration on Consciousness.[49][50][52][53]
Equity[edit | edit source]
To make global surgical care equitable, then surgical training (including simulation models) for the Global South must be equivalent in quality to the Global North. This module gives surgical practitioners in LMICs access to open-source, affordable, high fidelity, locally reproducible 3D printed bone simulation models that are comparable to state-of-the-art, quality-tested artificial bone products that are available in high income countries.[33][38]
To advance surgical education and care in LMICs, surgical simulation training must be standardized to monitor performance and clinical outcomes. Providing standardized surgical simulation training requires using reproducible simulators. This module provides high fidelity, 3D printed bone models that can be locally reproduced in any country with an inexpensive, single extruder, fused deposition modeling desktop 3D printer with a minimum build volume Z height of 210 mm, such as the open-source Original Prusa i3 MK3S+ that costs $999 USD.[24] This Appropedia module and our open-source, high fidelity, locally reproducible 3D printed bone simulators can empower unrestricted access to standardized, self-assessed orthopedic surgical simulation training around the world.
Targeted Feedback[edit | edit source]
The Tibial Shaft Simulator has a simulated Soft Tissue Layer for Plunge Depth Measurement to permit the measurement of plunge depth for the self-assessment framework.
The transparent cellophane that is wrapped around the Tibial Shaft Transverse Fracture Simulator and simulates the overlying periosteum will permit the learner to visually inspect and confirm that the self-drilling Schanz Screws did not perforate the far cortex for the self-assessment framework.
Unlike conventional artificial bone fracture models, the base of the 3D printed models of the Tibial Shaft Transverse Fracture Simulator allows the proximal and distal fracture fragments to be positioned on a flat surface to permit easy, convenient, and precise measurement of the drill trajectory angles of the Schanz Screws using a low-cost (20¢ USD) protractor for the self-assessment framework.[54][40]
Drawbacks of Traditional Approaches[edit | edit source]
According to the Global Surgical Training Challenge Discovery Awards: Mentoring Programme Handbook - March 2021, "anti-skills are clinically irrelevant skills that the simulator teaches that need to be unlearned when the clinician attempts to transfer what they have learned over to a real clinical scenario," and "[a]t its most fundamental level, anti-skills means incorrect or irrelevant technique, approach and methodology developed or reinforced as a result of using simulation."
During bicortical drilling, the surgeon adjusts drilling direction, speed and applied force to minimize plunge depth.[18] Drilling forces are influenced by cortical thickness, bone site, patient age, and bone mineral density.[19] Low fidelity simulators that do not accurately replicate human cortical bone hardness, and age- and gender-specific cortical thicknesses of tibial diaphyseal sites could foster anti-skills for bicortical drilling in learners. 100% of the orthopedic surgeons (n = 5) we surveyed agreed or strongly agreed that training on high fidelity simulators that accurately replicate human cortical bone hardness will translate into improved plunge depths when performing bicortical drilling on patients compared to low fidelity simulators.
Alternative approaches to orthopedic surgery simulation training include (in order of increasing fidelity): virtual reality simulators, DIY bone simulators, like the Fundamentals of Orthopedic Surgery (FORS) simulator, animal models, synthetic bones, and cadaver laboratories.[55][56]
Virtual reality simulators can be expensive and do not provide haptic or acoustic feedback for bicortical drilling skills training.
Low-cost DIY bone simulation models can be time-consuming to prepare and can vary widely in fidelity. This makes it impossible to provide standardized simulation training and to obtain objective, generalizable evaluation data to verify that the user's learnings will directly translate into the safe, clinical performance of bicortical drilling and external modular fixation procedures and will not foster the development of anti-skills.
The FORS simulator can be built from supplies purchased at a local hardware store.[56] However, the FORS Simulator uses low-fidelity polyvinyl chloride (PVC) hollow pipes which:
- do not recreate bone contours so the learner is unable to identify anatomic landmarks for proper positioning of external fixation pins into safe zones of the tibia, avoiding having the pins enter a joint cavity, or verifying post-fixation fracture alignment
- do not simulate cancellous bone
- are not available in accurate cortical thicknesses or cross-sectional shapes which could foster the development of anti-skills for bicortical drilling
- are made from vinyl chloride, an industrial carcinogen, and contain small amounts of phthalates, which are endocrine disrupting chemicals, and
- do not readily biodegrade and thus, contribute to the rising global levels of plastic waste in landfills, microplastics in oceans and atmosphere, and nanoplastics in the environment.[57][58][59][60][61][62][63][64][65][66][67]
Animal bones do not accurately simulate the length of human bones which would prevent learners from using the correctly sized orthopedic surgical hardware during simulation training.[68] Animal bones also do not have realistic human cortical thicknesses or cross-sectional shapes and this could foster the development of anti-skills for bicortical drilling.[69][70] Studies have shown significantly different drilling temperature values (which correlate with drilling energy and drilling force) in animal versus human bones.[19][69][70] Animal bones also require the use of specialized, costly vise clamps ($730.50 USD per pair) for securing the models for safe simulation-based training and the slaughtering of sentient beings and additional time-consuming preparation for use in simulation training and cannot reliably reproduce different fracture patterns to permit standardized orthopedic surgical skills training.[53][71][72]
Artificial bones are inaccessible in low to middle income countries due to long delivery times and high import costs from customs dues and processing fees because they are not produced locally.[33][38]
There is limited or no practitioner access to costly cadaver labs outside of training centers.
Acknowledgements[edit | edit source]
This work is funded by a grant from the Intuitive Foundation. Any research, findings, conclusions, or recommendations expressed in this work are those of the author(s), and not of the Intuitive Foundation.
References[edit | edit source]
- ↑ John A. Ruder, Blake Turvey, Joseph R. Hsu, Brian P. Scannell, Effectiveness of a Low-Cost Drilling Module in Orthopaedic Surgical Simulation, Journal of Surgical Education, Volume 74, Issue 3, 2017, Pages 471-476, ISSN 1931-7204, https://doi.org/10.1016/j.jsurg.2016.10.010.
- ↑ Efi Kazum, Oleg Dolkart, Yoav Rosenthal, Haggai Sherman, Eyal Amar, Moshe Salai, Eran Maman, Ofir Chechik, A Simple and Low-cost Drilling Simulator for Training Plunging Distance Among Orthopedic Surgery Residents, Journal of Surgical Education, Volume 76, Issue 1, 2019, Pages 281-285,ISSN 1931-7204, https://doi.org/10.1016/j.jsurg.2018.06.018.
- ↑ Bone Clamp [Internet]. Sawbones. [cited 2021 Dec 12]. Available from: https://www.sawbones.com/clamp-universal-w-c-clamp-1605.html.
- ↑ https://www.sawbones.com/clamp-vise-grip-used-in-conjunction-w-1600-or-1605-used-mainly-for-holding-irregularly-shaped.html
- ↑ Höntzsch D. Modular External Fixator [Internet]. AO Foundation. 2021 [cited 2021 Dec 11]. Available from: https://surgeryreference.aofoundation.org/orthopedic-trauma/adult-trauma/tibial-shaft/simple-fracture-transverse/modular-external-fixator#pin-insertion-tibial-shaft-.
- ↑ Raemer, D., Hannenberg, A. & Mullen, A. Simulation safety first: an imperative. Adv Simul 3, 25 (2018). https://doi.org/10.1186/s41077-018-0084-3.
- ↑ Ugochukwu EG, Ugbem LP, Ijomone OM, Ebi OT. Estimation of Maximum Tibia Length from its Measured Anthropometric Parameters in a Nigerian Population. J Forensic Sci Med [serial online] 2016 [cited 2021 Jun 27];2:222-8. Available from: https://www.jfsmonline.com/text.asp?2016/2/4/222/197928.
- ↑ U.S. Department of Health and Human Services — National Institutes of Health. Human tibia and fibula. [Internet]. Bethesda, (MD): NIH 3D Print Exchange; 2014 May 29 [cited 2021 Aug 17]. Available from: https://3dprint.nih.gov/discover/3DPX-000169.
- ↑ Gosman JH, Hubbell ZR, Shaw CN, Ryan TM. Development of cortical bone geometry in the human femoral and tibial diaphysis. Anat Rec (Hoboken). 2013 May;296(5):774-87. doi: 10.1002/ar.22688. Epub 2013 Mar 27. PMID: 23533061.
- ↑ 10.0 10.1 10.2 Ultimaker. Ultimaker PLA Technical Data Sheet [Internet]. Ultimaker Support. [cited 2021 July 29]. Available from: https://support.ultimaker.com/hc/en-us/articles/360011962720-UltimakerPLA-TDS.
- ↑ 11.0 11.1 Vian, Wei Dai and Denton, Nancy L., "Hardness Comparison of Polymer Specimens Produced with Different Processes" (2018). ASEE IL-IN Section Conference. 3. https://docs.lib.purdue.edu/aseeil-insectionconference/2018/tech/3.
- ↑ 12.0 12.1 Society For Biomaterials 30th Annual Meeting Transactions, page 332. Femoral Cortical Wall Thickness And Hardness Evaluation. K. Calvert, L.A. Kirkpatrick, D.M. Blakemore, T.S. Johnson. Zimmer, Inc., Warsaw, IN.
- ↑ Meyers, M. A.; Chen, P.-Y. (2014). Biological Materials Science. Cambridge: Cambridge University Press. ISBN 978-1-107-01045-1.
- ↑ Forrest AM, Johnson AE, inventors; Pacific Research Laboratories, Inc., assignee. Artificial bones and methods of making same. United States patent 8,210,852 B2. Date issued 2012 Jul 3.
- ↑ National Institutes of Health Osteoporosis and Related Bone Diseases National Resource Center. What is Bone? [Internet]. Bethesda (MD): The National Institutes of Health (NIH); 2018. [Cited 2021 Aug 17]. Available from: https://www.bones.nih.gov/health-info/bone/bone-health/what-is-bone.
- ↑ 16.0 16.1 16.2 16.3 Maeda K, Mochizuki T, Kobayashi K, Tanifuji O, Someya K, Hokari S, Katsumi R, Morise Y, Koga H, Sakamoto M, Koga Y, Kawashima H. Cortical thickness of the tibial diaphysis reveals age- and sex-related characteristics between non-obese healthy young and elderly subjects depending on the tibial regions. J Exp Orthop. 2020 Oct 6;7(1):78. doi: 10.1186/s40634-020-00297-9. PMID: 33025285; PMCID: PMC7538524.
- ↑ 17.0 17.1 Babalola OM, Salawu ON, Ahmed B A, Ibraheem G H, Olawepo A, Agaja S B. Epidemiology of traumatic fractures in a tertiary health center in Nigeria. J Orthop Traumatol Rehabil [serial online] 2018 [cited 2021 Jul 1];10:87-9. Available from: https://www.jotr.in/text.asp?2018/10/2/87/245994.
- ↑ 18.0 18.1 Khokhotva M, Backstein D, Dubrowski A. Outcome errors are not necessary for learning orthopedic bone drilling. Can J Surg. 2009 Apr;52(2):98-102. PMID: 19399203; PMCID: PMC2663499. URL: https://pubmed.ncbi.nlm.nih.gov/19399203/.
- ↑ 19.0 19.1 19.2 Pandey RK, Panda SS. Drilling of bone: A comprehensive review. J Clin Orthop Trauma. 2013 Mar;4(1):15-30. doi: 10.1016/j.jcot.2013.01.002. Epub 2013 Jan 18. PMID: 26403771; PMCID: PMC3880511.
- ↑ 20.0 20.1 Chen, J.V., Dang, A.B.C. & Dang, A. Comparing cost and print time estimates for six commercially-available 3D printers obtained through slicing software for clinically relevant anatomical models. 3D Print Med 7, 1 (2021). https://doi.org/10.1186/s41205-020-00091-4.
- ↑ 21.0 21.1 https://www.stratasys.com/en/3d-printers/printer-catalog/polyjet/j750-digital-anatomy-printer/?utm_source=google&utm_medium=cpc&utm_campaign=Stratasys-Polyjet-Brand&utm_term=stratasys%20j750&gclid=EAIaIQobChMI5tbD9oy99gIVOWxvBB0TcwsUEAAYAiAAEgKQ_PD_BwE
- ↑ https://www.3dlifeprints.com/products-services/simulation-training-phantom/
- ↑ https://www.3dlifeprints.com/products-services/anatomical-models/
- ↑ 24.0 24.1 Prusa J. Open-Source 3D printers from Josef Prusa [Internet]. Prusa3D. 2019 [cited 2021 July 29]. Available from: https://www.prusa3d.com/.
- ↑ Ultimaker. Ultimaker 3D printers: Reliable and easy to use [Internet]. ultimaker.com. [cited 2021 July 29]. Available from: https://ultimaker.com/3d-printers.
- ↑ Werz SM, Zeichner SJ, Berg BI, Zeilhofer HF, Thieringer F. 3D printed surgical simulation models as educational tool by maxillofacial surgeons. Eur J Dent Educ. 2018;22(3):e500–5. https://doi.org/10.1111/eje.12332.
- ↑ Legocki AT, Duffy-Peter A, Scott AR. Benefits and Limitations of Entry-Level 3-Dimensional Printing of Maxillofacial Skeletal Models. JAMA Otolaryngol Head Neck Surg. 2017;143(4):389–394. doi:10.1001/jamaoto.2016.3673
- ↑ Fargo Additive Manufacturing Equipment 3D, LLC. 3D printers, parts, and filament [Internet]. LulzBot. [cited 2021 July 29]. Available from: https://www.lulzbot.com/.
- ↑ Ultimaker. Ultimaker PLA. [Internet]. Utrecht (Netherlands): Ultimaker BV; 2021 [cited 2021 Aug 20]. Available from: https://ultimaker.com/materials/pla.
- ↑ Wong JY. 3D Printing Applications for Space Missions. Aerosp Med Hum Perform. 2016 Jun;87(6):580-582. doi: 10.3357/AMHP.4633.2016. PMID: 27208683.
- ↑ Filaments.ca. Value PLA 4043D Filament - White - 1.75mm - 1KG [Internet]. Filaments.ca. 2021 [cited 2021 Dec 10]. Available from: https://filaments.ca/collections/3d-filaments/products/value-pla-filament-white-1-75mm.
- ↑ 32.0 32.1 32.2 32.3 32.4 Kuunda 3D Ltd. Personal communication. July 14, 2021.
- ↑ 33.0 33.1 33.2 33.3 Sawbones. Tibia, Plastic Cortical Shell, Large - SKU:1104-9. [Internet]. Vashon, (WA): Sawbones; [cited 2021 Aug 26]. Available from: https://www.sawbones.com/tibia-large-left-solid-white-plastic-no-canal-1104-9.html.
- ↑ 34.0 34.1 Dr. Habila Umaru. Personal communication. May 13, 2021.
- ↑ Sawbones. Tibia with 12.5 mm Canal, Solid Foam, Left, Large [Internet]. Best Anatomical Medical Training Models Company. 2021 [cited 2021 Dec 11]. Available from: https://www.sawbones.com/tibia-large-left-solid-foam-w-canal1125.html.
- ↑ Best Orthopedic Workshop Bone Models for Surgical Skills Education training [Internet]. Sawbones; [cited 2021 Dec 10]. Available from: https://www.sawbones.com/orthopaedic-models-product-info.
- ↑ Sawbones. Cylinder with Encapsulated Oblique Fracture [Internet]. Best Anatomical Medical Training Models Company. Sawbones; 2021 [cited 2021 Nov 28]. Available from: https://www.sawbones.com/cylinder-short-oblique-fracture-w-single-neoprene-cover-1521-617-4.html.
- ↑ 38.0 38.1 38.2 38.3 38.4 38.5 Cylinder 40 mm OD x 6 mm wall, hollow, fourth generation [Internet]. Best Anatomical Medical Training Models Company. Sawbones; [cited 2021 Nov 28]. Available from: https://www.sawbones.com/cylinder-40mm-od-w-6mm-wall-length-500mm-4th-gen-composite3403-7.html.
- ↑ Sawbones. Cylinder 10 mm OD x 2 mm Wall, Hollow, Fourth Generation [Internet]. Best Anatomical Medical Training Models Company. Sawbones; 2021 [cited 2021 Nov 28]. Available from: https://www.sawbones.com/cylinder-10mm-od-w-2mm-wall-length-150mm-4th-gen-composite3403-17.html.
- ↑ 40.0 40.1 General Catalog - Sawbones [Internet]. Sawbones; [cited 2021 Nov 28]. Available from: https://www.sawbones.com/media/assets/product/documents/general_catalog.pdf.
- ↑ Recommendations For Labeling Medical Products To Inform Users That The Product Or Product Container Is Not Made with Natural Rubber Latex Guidance For Industry And Food and Drug Administration Staff [Internet]. United States Food and Drug Administration; 2014 [cited 2021 Nov 28]. Available from: https://www.fda.gov/media/85473/download.
- ↑ Liu, K., Madbouly, S. A., Schrader, J. A., Kessler, M. R., Grewell, D. and Graves, W. R. (2015), Biorenewable polymer composites from tall oil-based polyamide and lignincellulose fiber. J. Appl. Polym. Sci., 132, 42592. doi: 10.1002/app.42592.
- ↑ Mills, C. A.; Navarro, M.; Engel, E.; Martinez, E.; Ginebra, M. P.; Planell, J.; Errachid, A.; Samitier, J. J. Biomed. Mater. Res. A 2006, 76A, 781.
- ↑ Auras, R.; Harte, B.; Selke, S. Macromol. Biosci. 2004, 4, 835.
- ↑ Chemical Composition and Toxicity of Particles Emitted from a Consumer-Level 3D Printer Using Various Materials. Qian Zhang, Michal Pardo, Yinon Rudich, Ifat Kaplan-Ashiri, Jenny P. S. Wong, Aika Y. Davis, Marilyn S. Black, and Rodney J. Weber. 2019 53 (20), 12054-12061. DOI: 10.1021/acs.est.9b04168.
- ↑ Emissions of Ultrafine Particles and Volatile Organic Compounds from Commercially Available Desktop Three-Dimensional Printers with Multiple Filaments. Parham Azimi, Dan Zhao, Claire Pouzet, Neil E. Crain, and Brent Stephens. 2016 50 (3), 1260-1268. DOI: 10.1021/acs.est.5b04983.
- ↑ Ultimaker. 3D printer emissions and indoor air quality -White paper. [Internet]. Utrecht (Netherlands): Ultimaker BV; 2019 Oct [cited 2021 Aug 17]. Available from: https://ultimaker.com/learn/3d-printer-emissions-and-indoor-air-quality.
- ↑ Wikipedia. Hinduism. [Internet]. San Francisco (CA): Wikipedia; 2021 [cited 2021 Aug 18]. Available from: https://en.wikipedia.org/wiki/Hinduism.
- ↑ 49.0 49.1 Wikipedia. Buddhism. [Internet]. San Francisco (CA): Wikipedia; 2021 [cited 2021 Aug 18]. Available from: https://en.wikipedia.org/wiki/Buddhism.
- ↑ 50.0 50.1 Wikipedia. Ahimsa in Jainism. [Internet]. San Francisco (CA): Wikipedia; 2021 [cited 2021 Aug 18]. Available from: https://en.wikipedia.org/wiki/Ahimsa_in_Jainism.
- ↑ Wikipedia. Veganism. [Internet]. San Francisco (CA): Wikipedia; 2021 [cited 2021 Aug 18]. Available from:https://en.wikipedia.org/wiki/Veganism#cite_note-26.
- ↑ Wikipedia. Ahimsa. [Internet]. San Francisco (CA): Wikipedia; 2021 [cited 2021 Aug 18]. Available from: https://en.wikipedia.org/wiki/Ahimsa.
- ↑ 53.0 53.1 Low P. The Cambridge Declaration on Consciousness. In: Jaak Panksepp J, Reiss D, Edelman D, Swinderen BV, Low P, and Koch C, editors. The Francis Crick Memorial Conference - Consciousness in Humans and Non-Human Animals; 2012 July 7; Cambridge, UK. Available from: https://fcmconference.org/img/CambridgeDeclarationOnConsciousness.pdf.
- ↑ Staples Canada. Acme 4" Plastic Protractor [Internet]. Staples. 2021 [cited 2021 Dec 11]. Available from: https://www.staples.ca/products/38953-en-acme-4-plastic-protractor.
- ↑ Osso VR. Osso VR. [Internet]. San Francisco (CA): Osso VR; 2021 [cited 2021 Aug 18]. Available from: https://www.ossovr.com/.
- ↑ 56.0 56.1 Lopez G, Wright R, Martin D, Jung J, Bracey D, Gupta R. A cost-effective junior resident training and assessment simulator for orthopaedic surgical skills via fundamentals of orthopaedic surgery: AAOS exhibit selection. J Bone Joint Surg Am. 2015 Apr 15;97(8):659-66. DOI: https://doi.org/10.2106/JBJS.N.01269.
- ↑ Fralish MS, Downs JW. Vinyl Chloride Toxicity. [Updated 2021 Jun 29]. In: StatPearls [Internet]. Treasure Island (FL): StatPearls Publishing; 2021 Jan-. Available from: https://www.ncbi.nlm.nih.gov/books/NBK544334/.
- ↑ CDC. National Biomonitoring Program - Phthalates Factsheet. [Internet]. Atlanta (GA): CDC; Last reviewed 2021 Apr 5 [cited 2021 Aug 17]. Available from: https://www.cdc.gov/biomonitoring/Phthalates_FactSheet.html.
- ↑ Geyer, R., Jambeck, J.R. and Law, K.L., 2017. Production, use, and fate of all plastics ever made. Science advances, 3(7), p.e1700782.
- ↑ Tang, C.C., Chen, H.I., Brimblecombe, P. and Lee, C.L., 2018. Textural, surface and chemical properties of polyvinyl chloride particles degraded in a simulated environment. Marine pollution bulletin, 133, pp.392-401.
- ↑ Veronelli, M., Mauro, M. and Bresadola, S., 1999. Influence of thermal dehydrochlorination on the photooxidation kinetics of PVC samples. Polymer degradation and stability, 66(3), pp.349-357.
- ↑ Guo, J.J., Huang, X.P., Xiang, L., Wang, Y.Z., Li, Y.W., Li, H., Cai, Q.Y., Mo, C.H. and Wong, M.H., 2020. Source, migration and toxicology of microplastics in soil. Environment international, 137, p.105263.
- ↑ O'Connor, D., Pan, S., Shen, Z., Song, Y., Jin, Y., Wu, W.M. and Hou, D., 2019. Microplastics undergo accelerated vertical migration in sand soil due to small size and wet-dry cycles. Environmental Pollution, 249, pp.527-534.
- ↑ Hidalgo-Ruz, V., Gutow, L., Thompson, R.C. and Thiel, M., 2012. Microplastics in the marine environment: a review of the methods used for identification and quantification. Environmental science & technology, 46(6), pp.3060-3075.
- ↑ Auta, H.S., Emenike, C.U. and Fauziah, S.H., 2017. Distribution and importance of microplastics in the marine environment: a review of the sources, fate, effects, and potential solutions. Environment international, 102, pp.165-176.
- ↑ Wright, S.L., Ulke, J., Font, A., Chan, K.L.A. and Kelly, F.J., 2020. Atmospheric microplastic deposition in an urban environment and an evaluation of transport. Environment international, 136, p.105411.
- ↑ Wang, L., Wu, W.M., Bolan, N.S., Tsang, D.C., Li, Y., Qin, M. and Hou, D., 2021. Environmental fate, toxicity and risk management strategies of nanoplastics in the environment: Current status and future perspectives. Journal of hazardous materials, 401, p.123415.
- ↑ Encinas-Ullán CA, Martínez-Diez JM, Rodríguez-Merchán EC. The use of external fixation in the emergency department: applications, common errors, complications and their treatment. EFORT Open Rev. 2020 Apr 2;5(4):204-214. doi: 10.1302/2058-5241.5.190029. PMID: 32377388; PMCID: PMC7202044.
- ↑ 69.0 69.1 Eriksson AR, Albrektsson T, Albrektsson B. Heat caused by drilling cortical bone. Temperature measured in vivo in patients and animals. Acta Orthop Scand. 1984;55(6):629e631.
- ↑ 70.0 70.1 Hillery HT, Shuaib I. Temperature effects in drilling of human and bovine bone. J Mater Process Technol. 1999;92e93: 302e308.
- ↑ https://www.sawbones.com/clamp-vise-grip-used-in-conjunction-w-1600-or-1605-used-mainly-for-holding-irregularly-shaped.html
- ↑ https://www.sawbones.com/clamp-universal-w-c-clamp-1605.html